The scientists working on Planck have been awarded a Physics World Top 10 breakthrough of 2013 “for making the most precise measurement ever of the cosmic microwave background radiation”.
The European Space Agency’s Planck satellite is the first European mission to study the origins of the universe. It surveyed the microwave sky from 2009 to 2013, measuring the cosmic microwave background (CMB), the afterglow of the Big Bang, and the emission from gas and dust in our own Milky Way galaxy. The satellite performed flawlessly, yielding a dramatic improvement in resolution, sensitivity, and frequency coverage over the previous state-of-the-art full sky CMB dataset, from NASA’s Wilkinson Microwave Anisotropy Probe.
The first cosmology data from Planck was released in March 2013, containing results ranging from a definitive picture of the primordial fluctuations present in the CMB temperature, to a new understanding of the constituents of our Galaxy. These results are due to the extensive efforts of hundreds of scientists in the international Planck Collaboration. Here we focus on critical contributions made at UCL to these results.
Planck’s detectors can measure temperature differences of millionths of a degree. To achieve this, some of Planck‘s detectors must be cooled to about one-tenth of a degree above absolute zero – colder than anything in nature – so that their own heat does not swamp the signal from the sky. Giorgio Savini spent the five years prior to launch building the cold lenses as well as testing and selecting all the other optical components which constitute the “eyes” of the Planck High Frequency Instrument. During the first few months of the mission he helped to analyze the data to make sure that the measurements taken in space and the calibration data on the ground were consistent.
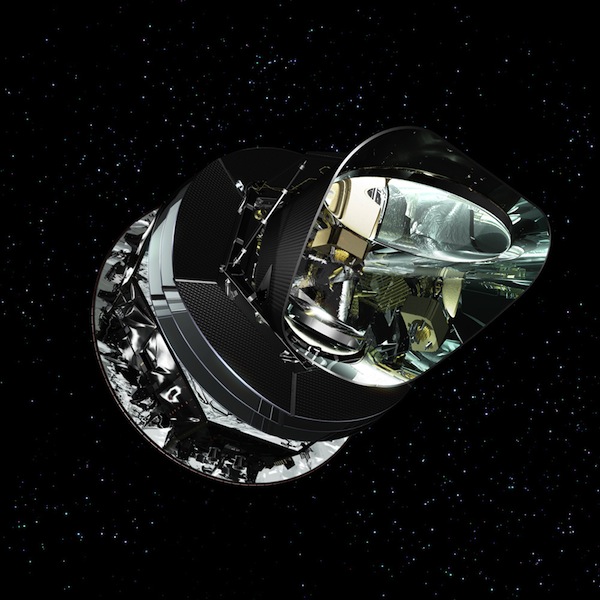
Artist’s rendering of the Planck satellite with view inside the telescope shields. The focal plane unit is visible as the golden collection of waveguide horns at the focus of the telescope positioned inside the thermal shields (external envelope) which protect the telescope from unwanted stray light and aids the cooling of the telescope mirrors by having a black emitting surface on the outside and a reflective one on the inside. For reference, the Earth and Sun would be located far towards the bottom left of this picture. (Credit: ESA/Planck)
UCL researchers Hiranya Peiris, Jason McEwen, Aurélien Benoit-Lévy and Franz Elsner played key roles in using the Planck cosmological data to understand the origin of cosmic structure in the early universe, the global geometry and isotropy of the universe, and the mass distribution of the universe as traced by lensing of the CMB. As a result of Planck’s 50 megapixel map of the CMB, our baby picture of the Universe has sharpened, allowing the measurement of the parameters of the cosmological model to percent precision. As an example, Planck has measured the age of the universe, 13.85 billion years, to half per-cent precision.

The anisotropies of the cosmic microwave background (CMB) as observed by Planck. The CMB is a snapshot of the oldest light in our Universe, imprinted on the sky when the Universe was just 380 000 years old. It shows tiny temperature fluctuations that correspond to regions of slightly different densities, representing the seeds of all future structure: the stars and galaxies of today. (Credit: ESA/Planck)
The precision of the measurements has also allowed us to rewind the story of the Universe back to just a fraction of a second after the Big Bang. At that time, at energies about a trillion times higher than produced by the Large Hadron Collider at CERN, all the structure in the Universe is thought to have been seeded by the quantum fluctuations of a so-called scalar field, the inflaton. This theory of inflation predicts that the power in the CMB fluctuations should be distributed as a function of wavelength in a certain way. For the first time, Planck has detected, with very high precision, that the Universe has slightly more power on large scales compared with small scales – the cosmic symphony is very slightly bass-heavy, yielding a key clue to the origin of structure in the Universe. Inflation also predicts that the CMB fluctuations will have the statistical properties of a Gaussian distribution. Planck has verified this prediction to one part in 10,000 – this is the most precise measurement we have in cosmology.
As the CMB photons travel towards us their paths get very slightly bent by massive cosmological structures, like clusters of galaxies, that they have encountered on the way. This effect, where the intervening (dark) matter acts like a lens – only caused by gravity, not glass – on the photons, slightly distorts the CMB. The Planck team was able to analyse these distortions, extract the lensing signature in the data, and create the first full-sky map of the entire matter distribution in our Universe, through 13 billion years of cosmic time. A new window on the cosmos has been opened up.
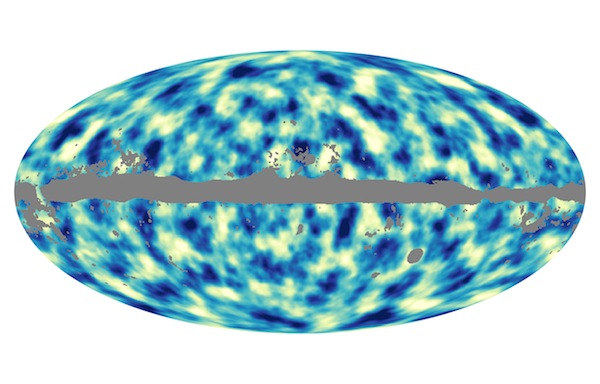
This all-sky image shows the distribution of dark matter across the entire history of the Universe as seen projected on the sky. It is based on data collected with ESA’s Planck satellite during its first 15.5 months of observations. Dark blue areas represent regions that are denser than the surroundings, and bright areas represent less dense regions. The grey portions of the image correspond to patches of the sky where foreground emission, mainly from the Milky Way but also from nearby galaxies, is too bright, preventing cosmologists from fully exploiting the data in those areas. (Credit: ESA/Planck)
Einstein’s theory of general relativity tells us about the local curvature of space-time but it cannot tell us about the global topology of the Universe. It is possible that our Universe might have a non-trivial global topology, wrapping around itself in a complex configuration. It is also possible that our Universe might not be isotropic, i.e., the same in all directions. The exquisite precision of Planck data allowed us to put such fundamental assumptions to the test. The Planck team concluded that our Universe must be close to the standard topology and geometry, placing tight constraints on the size of any non-trivial topology; some intriguing anomalies remain at the largest observable scales, requiring intense analysis in the future.
Aside from additional temperature data not included in the first year results, the upcoming Planck data release in summer 2014 will also include high resolution full-sky polarization maps. This additional information will not only allow us to improve our measurements of the cosmological parameters even further; it will also advance our understanding of our own Galaxy by probing the structure of its magnetic fields and the distribution and composition of dust molecules. There is a further, exciting possibility: if inflation happened, the structure of space should be ringing with primordial gravitational waves, which can be detected in the polarized light of the CMB. We may be able to detect these in the Planck polarization data.
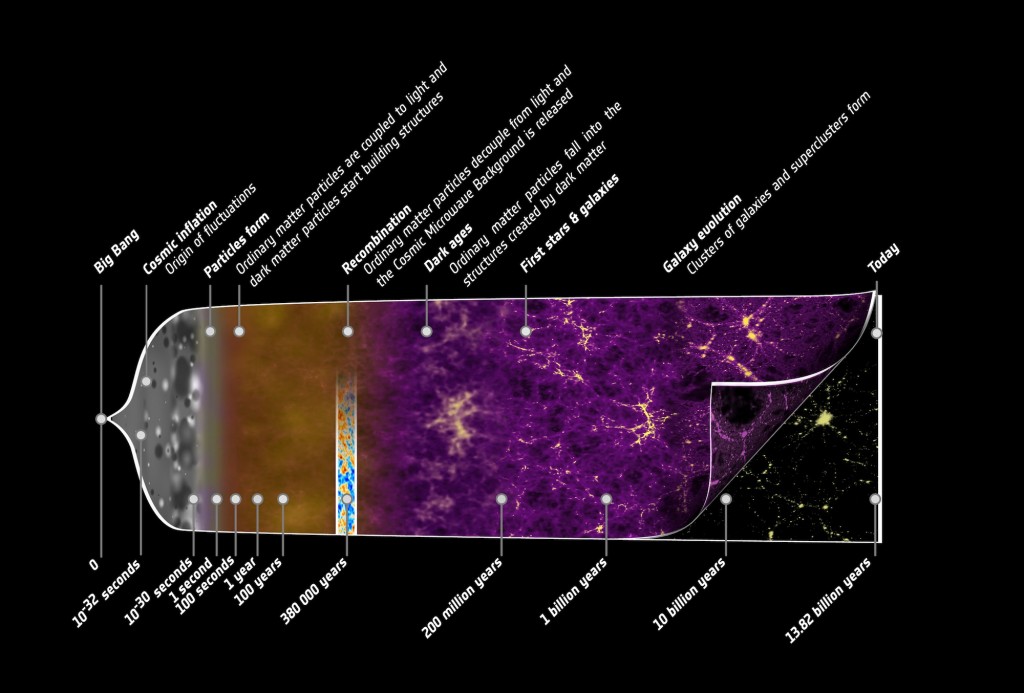
This illustration summarises the almost 14-billion-year long history of our Universe. It shows the main events that occurred between the initial phase of the cosmos, where its properties were almost uniform and punctuated only by tiny fluctuations, to the rich variety of cosmic structure that we observe today, from stars and planets to galaxies and galaxy clusters. (Credit: ESA/Planck)